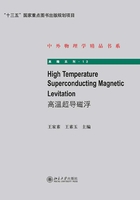
2.7 Trapped fluxes in HTS bulk
The rare-earth PMs with high energy density and high intrinsic coercive force are widely used, bringing great technical and economic benefit. Superconductors can trap a high magnetic flux and thereby can become a PM.The superconducting PMs have much higher energy density than the rare-earth PMs, and their potential industrial applications are very promising.
Trapped fields in LTS bulk Nb3Sn were investigated at the temperature of 4. 2 K(liquid helium)to 1.5 K in 1965.A Nb3Sn hollow cylinder,18.6 mm long,10.2 mm outer diameter, and 2.7 mm inside diameter, was synthesized by combines niobium tin powder and sintering technique.The sintered bulk Nb3Sn hollow cylinder had demonstrated that a magnetic field of 0.2 T,0.4 T, and 2.7 T can be trapped at 4.2 K,2.2 K, and l.5 K, respectively150.
In 1977,a hollow Nb3Sn superconducting cylinder of 2. 45 cm in diameter was used to trap a magnetic field of 2.24 T at 4.2 K151.The cylinder of 7.62 cm was constructed by helically wrapping 44 layers of superconducting Nb3Sn ribbon 2.54 cm wide by 75μm thick around a mandrel.
However, the trapped field in LTS bulk superconductors at low temperatures was found to be strongly limited by thermomagnetic instabilities, resulting fromflux jumps as the external field exceeds certain values of the applied magnetic field. Due to the low specific heat of LTS materials in superconducting state, the thermomagnetic instabilities are very pronounced at low temperatures(see section 2.5.1).
In contrast to LTS materials, there are some peculiar features in the specific heat of YBCO compared with those of conventional BCS superconductors, namely HTS bulks are thermally stable even in large sample sizes due to their relatively large specific heat in the superconducting state. Due to the larger specific heat of HTSC, the heat within the superconductor is released to the surrounding cryogen.Therefore, thermal instabilities have no influence on the trapped field in HTSC, at least at temperatures above 30 K.Very high trapped fields can be achieved by the HTS bulk material.The superconducting PM and its applications have become a reality because of HTSC material.
In engineering applications, high texture and c-axis-orientated single domains are required. Large-sized, high-performance REBCO single domains are now com mercially available.The trapped flux density(Btrap )due to flux pinning and the associated superconducting currents flowing persistently in a REBCO can be ex pressed in a simple model, as152

where A is a geometrical constant,μ0 is the permeability of the vacuum, and r is the radius of the grain.In order to increase the trapped flux, both the critical current density, the dimension and the homogeneity of HTSC bulk must be enhanced.Increase of the critical current density is accomplished by the improvement of flux pinning properties.

where A is constant, Vf is the volume fraction and d is the average diameter of Y211 particles. Thus, under constant volume of the second phase particles, Jc is inversely proportional to the size of the Y211 particles153.
In order to avoid the influence of a temperature rise on thermal stability, the cooling power of the system must be larger than local heat generation. Since the thermal conductivity of HTS bulk is small(see section 2.5.2,about 2-10 W/mK at77 K),cooling by cryogen at the bulk's surface is not sufficient to cool the interior region, leading to a local temperature rise.The temperature rise will reduce the flux pinning capability and induced flux jumps.Once the flux jumps appear in a HTS bulk, it is no longer stable.When the flux jump is serious enough, the HTS bulk will be destroyed due to the crack of formation from the large electromagnetic forces.Thus for the superconducting PMs, it is very important to maintain the thermal stability.
These are serious challenges for superconducting materials experts. It is nec essary to prevent the generation of micro-cracks and micro-pores during the melt processing.There are some methods to improve the HTS bulk performance, for example, addition of silver, sample reinforcement with metal rings, etc.Tomita and Murakami have developed a post-fabrication treatment, which improves the mechanical properties as well as the thermal conductivity of a YBCO bulk magnet, thereby increasing its field-trapping capacity.These methods use the resin impreg nation and wrap the materials in carbon fiber, and also include a small hole drilled into the centre of the magnet to allow the impregnation of BiPbSnCd alloy into the superconductor.These methods greatly enhance the thermal stability and internal mechanical strength.As a result,17.24 T could be trapped, without fracturing, in a bulk Y-Ba-Cu-O sample of 2.65 cm diameter at 29 K(Fig.2.7.1)110.
There are three typical ways to magnetize a HTS bulk:field cooling(FC)mag netization, zero field cooling(ZFC)magnetization and pulse field magnetization(PFM). When the HTS bulk is magnetized in a magnetic field, the HTS bulk can trap flux.After removing the field, a superconducting permanent magnet can be obtained.In comparison, the ZFC magnetization is more difficult, because a very high external magnetic field is generally needed.The FC magnetization is conve nient.The applied magnetic field needs to reach at least somewhat above the level of the residual magnetic field.
The magnetization process may lead to a local temperature rise in superconduc tors. Oka et al.154reported this warming effect.The temperature rise of a SmBCO single-domain bulk superconductor was precisely measured during the ZFC pro cess.The highest temperature rise was reported as 7.5 K when the sample was magnetized at a sweeping rate of 5.06 m T s−1at 50 K.

Fig.2.7.1 The effect of temperature on trapped-field distribution.The field was trapped between two 26.5-mm-diameter YBCO disks for 29 K,46 K and 78 K110.
The Pulsed Field Magnetization(PFM)is considered to be the most practical method because it is inexpensive, small-volume and mobile experimental set-up to apply a magnetic field. However, the field trapped by PFM was smaller than that attained by FCM below 77 K, because of the large temperature rise caused by the magnetic flux motion in the bulk.In order to improve the magnetisation performance of HTS PM using PFM method, much work has been done.Sev eral approaches were tried and succeeded in enhancing trapped flux around 77 K, including such as iteratively magnetizing pulsed field operation with reducing am plitudes(IMRA)155and multi-pulse technique with stepwise cooling(MPSC)156.A single pulse magnetization gives a poor result compared to the ZFC, IMRA and MPSC magnetization.The trapped flux of the latter can be made to be almost as high as for the ideal field cooled case.
Fujishiro et al.157succeeded in establishing a record of high-field trapping, Btrap =5. 20T on theφ45 mm GdBCO bulk, using a 6.7 T applied field at 29 K.A maximum Btrap of 3.6 T was attained by a single pulse application.
Trapped field profiles for a 26 mm diameter GdBCO bulk magnetized at 77K in FCM and PFM were investigated158. FCM trapped a higher peak field(0.90 T)than PFM(0.63 T),but using IMRA allows pulsed magnetization to give approximately 95%of the field cooled trapped flux.The real effectiveness of PFM came when using both the IMRA and MPSC together.
In general, the pulsed magnetic field is provided by the solenoid in PFM. The pulsed field of the solenoid is not uniform and imposes the inhomogeneous pulsed-field distribution upon the magnetization of HTS bulk samples.Ida et al.159studied the magnetization of GdBCO which was inserted between two disks as pulsed-current vortex type Cu coils.As a result, the pulse current was smaller and magnetic field distribution was more uniform than for a solenoid tape.
Deng160presented experimental results for different penetration patterns of magnetic flux when employing vortex-type coils in PFM, that is, the magnetic flux will primarily penetrate inside the bulk from the upper and lower surfaces using vortex-type coils.In general, the size and current of vortex-type coils is smaller than that of a solenoid, so a reduced heat generation and temperature rise can be obtained by vortex coils, which is very important for PFM applications.
A new compact NMR magnet using HTS bulk magnets has been developed. However, it is difficult to trap a uniform magnetic field above 4.7 T at liquid nitrogen temperature.Kim et al.161presented a remagnetization method in order to improve the field homogeneity of a HTS bulk NMR relaxometry device, and obtained the 1.58 T and 9 ppm/cm3using the single HTS bulk with 20 mm inner diameter,60 mm outer diameter and 80 mm height by the remagnetization process.
Durrell et al. reported a trapped field of 17.6 T162in a stack of two GdBCO HTS bulks from a 17.8 T magnetizing field at 26 K.This is the highest field trapped in a bulk superconductor reported to date.The silver-doped GdBCO bulks were 25 mm in diameter, and fabricated by top-seeded melt growth and reinforced with shrink-fit stainless steel.
LTS bulks show large and sharp magnetic flux jumps that prevent their use. HTS bulks show higher thermal stability and can be used as superconducting PMs.The MgB2 is a new type of LTSCs.The MgB2 bulk has also a promising potential as superconducting PM.It has several attractive features for bulk magnets, suchas low-cost, light-weight and a homogeneous trapped field distribution.In addi-tion, flux jumps in MgB2 arise at low temperature(4.2 K),but tend to disappear at temperatures larger than 10 K.Perimi et al.20 have studied the magnetic field trapping capability of MgB2 discs of different shapes, at temperatures 10 K.The SIMS(superconductive inserts in metallic substrates)devices showed a higher sta bility of the trapped fields with respect to the bulk discs.Typical trapped fields, measured at 1 mm from the surface of the device, are of the order of 1 T.
Fujishino et al.24performed PFM on a large MgB2 bulk 50 mm in diameter, in which a trapped field Btrap =0. 47 T was achieved at 23 K.Thereafter, they reported a maximum trapped field Btrap =0.71 T which was realized at the center of the bulk surface at 14 K after a magnetic pulse application of Bex =1.55 T24.They found that the flux dynamics and the heat generation in a MgB2 bulk during PFM were clearly in contrast to those for REBCO bulks, because of the small specific heat, large thermal conductivity, and narrow temperature margin against Tc in the MgB2 bulk.
In order to reduce the cost and meet the needs of more and more applications, batch production of bulk HTSC been improved. Plechacek et al.118 have reported development and successful tests of a melt-powder-melt-growth process capable of simultaneous fabrication of up to 64 YBCO levitation disks, a necessary step towards the planned production capacity of several thousand pieces a year.Diam-eters of the HTS bulks varied from 14 mm to 56 mm.The trapped magnetic field measured at 77 K on the bulks arbitrarily chosen from different batches was in all cases higher than 0.5 T.Levitation force at 77 K with a levitation gap of 1 mm reached the same value of 80 N in all samples.The self-field critical current density Jc at 77 K exhibited values between 50 and 90 kA/cm2 at the bulk center and 70-95 kA/2 at the bulk edge.
A production run of 60 melt-textured YBCO trapped field magnets,2 cm in diameter, was reported by Sawh et al.163. The HTS PMs have pinning centers of Y211 and Pt0.4 U0.6 /YBa3 O6 deposits and also damage tracks due to ions from uranium fission.The resulting average trapped field at the center of the seed-side surface is 2.04 T at 77 K.
Trapped flux can be measured by magnetic sensor scanning, which will beintroduced in chapter 5.
HTS PM can be widely used in construction of practically frictionless bearings, non-contact spinners, Maglev flywheel energy storage devices, Maglev trains, motors, wind or tidal power generators, NMR/MRI, magnetic drag delivery, magnetic separation, magnetron sputtering, and contact-less liquid pumps, etc.