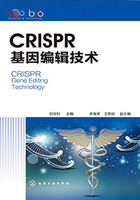
参考文献
[1] Thomas K R, Folger K R, Capecchi M R. High frequency targeting of genes to specific sites in the mammalian genome. Cell, 1986, 44(3):419-428.
[2] Kim Y G, Cha J, Chandrasegaran S. Hybrid restriction enzymes: zinc finger fusion to FokI cleavage domain. Proc Natl Acad Sci USA, 1996, 93(3): 1156-1160.
[3] Rahimov F, Kunkel L M. The cell biology of disease: cellular and molecular mechanisms underlying muscular dystrophy. J Cell Biol, 2013, 201(4):499-510.
[4] Ruszczak C, Mirza A, Menhart N. Differential stabilities of alternative exon-skipped rod otifs of dystrophin. Biochim Biophys Acta, 2009, 1794(6):921-928.
[5] Lu Q L, Yokota T, Takeda S, et al. The status of exon skipping as a therapeutic approach to Duchenne muscular dystrophy. Mol Ther, 2010, 19(1):9-15.
[6] Ran F A, Hsu P D, Jason Wright J, et al. Genome engineeringusing the CRISPR-Cas9 system. Nat Protoc, 2013, 8(11): 2281-2308.
[7] Ishino Y, Shinagawa H, Makino K. Nucleotide sequence of the iap gene, responsible for alkaline phosphatase isozyme conversion in Escherichia coli, and identification of the gene product. J Bacteriol, 1987, 169(12): 5429-5433.
[8] Barrangou R, Fremaux C, Deveau H. CRISPR provides acquired resistance against viruses in prokaryotes. Science, 2007, 315(5819): 1709-1712.
[9] Jinek M, Chylinski K, Fonfara I. A programmable dual-RNAguided DNA endonuclease in adaptive bacterial immunity. Science, 2012, 337(6096): 816-821.
[10] Deltcheva E, Chylinski K, Sharma C M. CRISPR RNA maturation by trans-encoded small RNA and host factor RNase Ⅲ. Nature, 2011, 471(7340): 602-607.
[11] Makarova K S, Wolf Y I, Alkhnbashi O S. An updated evolutionary classification of CRISPR-Cas systems. Nat Rev Microbiol, 2015, 13(11): 722-736.
[12] Shmakov S, Abudayyeh O O, Makarova K S. Discovery and functional characterization of diverse class 2 CRISPR-Cas systems. Mol Cell, 2015, 60(3): 385-397.
[13] Gasiunas G, Barrangou R, Horvath P. Cas9-crRNA ribonucleoprotein complex mediates specific DNA cleavage for adaptive immunity in bacteria. Proc Natl Acad Sci USA, 2012, 109(39): E2579-2586.
[14] Mohanraju P, Makarova K S, Zetsche B. Diverse evolutionary roots and mechanistic variations of the CRISPR-Cas systems. Science, 2016, 353(6299): aad5147.
[15] Agari Y, Sakamoto K, Tamakoshi M. Transcription profile of Thermus thermophilus CRISPR systems after phage infection. J Mol Biol, 2010, 395(2): 270-281.
[16] Kunin V, Sorek R, Hugenholtz P. Evolutionary conservation of sequence and secondary structures in CRISPR repeats. Genome Biol, 2007, 8(4): R61.
[17] van der Oost J, Westra E R, Jackson R N. Unravelling the structural and mechanistic basis of CRISPR-Cas systems. Nat. Rev. Microbiol, 2014, 12:479-492.
[18] Abudayyeh O O, Gootenberg J S, Konermann S. C2c2 is a single-component programmable RNA-guided RNA-targeting CRISPR effector. Science, 2016, 353(6299): aaf5573.
[19] Liu L, Li X, Ma J. The molecular architecture for RNA-guided RNA cleavage by Cas13a. Cell, 2017, 170(4): 714-726.
[20] Smargon A A, Cox D B T, Pyzocha N K. Cas13b is a type VI-B CRISPR-associated RNA-guided RNase differentially regulated by accessory proteins Csx27 and Csx28. Mol Cell, 2017, 65(4): 618-630.
[21] Makarova K S, Wolf Y I, Alkhnbashi O S. An updated evolutionary classification of CRISPR-Cas systems. Nat Rev Microbiol, 2015, 13(11): 722-736.
[22] Jiang Y, Bikard D, Cox D. RNA-guided editing of bacterial genomes using CRISPR-Cas systems. Nat Biotechnol, 2013, 31(3): 233-239.
[23] Wang Y, Zhang Z T, Seo S O. Markerless chromosomal gene deletion in Clostridium beijerinckii using CRISPR-Cas9 system. J Biotechnol, 2015, 200: 1-5.
[24] Wang Y, Zhang Z T, Seo S O. Bacterial genome editing with CRISPR-Cas9: deletion, integration, single nucleotide modification, and desirable “Clean” mutant selection in Clostridium beijerinckii as an example. ACS Synth Biol, 2016, 5(7): 721-732.
[25] Tong Y J, Charusanti P, Zhang L X. CRISPR-Cas9 based engineering of Actinomycetal genomes. ACS Synth Biol, 2015, 4(9): 1020-1029.
[26] Cobb R E, Wang Y J, Zhao M H. High-efficiency multiplex genome editing of Streptomyces species using an engineered CRISPR-Cas system. ACS Synth Biol, 2015, 4(6): 723-728.
[27] Xu T, Li Y C, Shi Z. Efficient genome editing in Clostridium cellulolyticum via CRISPR-Cas9 nickase. Appl Environ Microbiol, 2015, 81(13): 4423-4431.
[28] Penewit K, Holmes E A, Mclean K. Efficient and scalable precision genome editing in Staphylococcus aureus through conditional recombineering and CRISPR-Cas9-mediated counterselection. mBio, 2018, 9(1): e00067-18.
[29] Liu J, Wang Y, Lu Y J. Development of a CRISPR-Cas9 genome editing toolbox for Corynebacterium glutamicum. Microb Cell Fact, 2017, 16(1): 205.
[30] Jiang Y, Qian F H, Yang J J. CRISPR-Cpf1 assisted genome editing of Corynebacterium glutamicum. Nat Commun, 2017, 8: 15179.
[31] Zheng X, Li S Y, Zhao G P. An efficient system for deletion of large DNA fragments in Escherichia coli via introduction of both Cas9 and the non-homologous end joining system from Mycobacterium smegmatis. Biochem Biophys Res Commun, 2017, 485(4): 768-774.
[32] Chen W Z, Zhang Y, Zhang Y F. CRISPR-Cas9-based genome editing in Pseudomonas aeruginosa and cytidine deaminase-mediated base editing in Pseudomonas species. iScience, 2018, 6: 222-231.
[33] Zheng K, Wang Y, Li N. Highly efficient base editing in bacteria using a Cas9-cytidine deaminase fusion. Commun Biol, 2018, 1: 32-26.
[34] Wang Y, Liu Y, Liu J. MACBETH: Multiplex automated Corynebacterium glutamicum base editing method. Metab Eng, 2018, 47: 200-210.
[35] DiCarlo J E, Norville J E, Mali P. Genome engineering in Saccharomyces cerevisiae using CRISPR-Cas systems. Nucleic Acids Res. , 2013, 41(7): 4336-4343.
[36] Bao Z, Xiao H, Liang J. Homology-integrated CRISPR-Cas(HI-CRISPR)system for one-step multigene disruption in Saccharomyces cerevisiae. ACS Synth Biol, 2015, 4(5): 585-594.
[37] Jacobs J Z, Ciccaglione K M, Tournier V. Implementation of the CRISPR-Cas9 system in fission yeast. Nat Commun, 2014(5): 5344-5348.
[38] Shapiro R S, Chavez A, Porter C B M. A CRISPR-Cas9-based gene drive platform for genetic interaction analysis in Candida albicans. Nat. Microbiol, 2018, 3(1): 73-82.
[39] Liu R, Chen L, Jiang Y. Efficient genome editing in filamentous fungus Trichoderma reesei using the CRISPR /Cas9 system. Cell Discov, 2015(1): 15007-15017.
[40] Katayama T, Tanaka Y, Okabe T. Development of a genome editing technique using the CRISPR-Cas9 system in the industrial filamentous fungus Aspergillus oryzae. Biotechnol. Lett, 2016, 38(4): 637-642.
[41] Zhang C, Meng X, Wei X. Highly efficient CRISPR mutagenesis by microhomology- mediated end joining in Aspergillus fumigatus. Fungal Genet Biol, 2016(86): 47-57.
[42] Arazoe T, Miyoshi K, Yamato T. Tailor-made CRISPR-Cas system for highly efficient targeted gene replacement in the rice blast fungus. Biotechnol. Bioeng, 2015, 112(12): 2543-2549.
[43] Schuster M, Schweizer G, Reissmann S. Genome editing in Ustilago maydis using the CRISPR-Cas system. Fungal Genet Biol, 2016(9): 3-9.
[44] Waltz E. Gene-edited CRISPR mushroom escapes US regulation. Nature, 2016, 532(7599): 293.
[45] Sugano S S, Suzuki H, Shimokita E. Genome editing in the mushroom-forming basidiomycete Coprinopsis cinerea, optimized by a high- throughput transformation system. Sci Rep, 2017, 7(1): 1260-1268.
[46] Qin H, Xiao H, Zou G. CRISPR-Cas9 assisted gene disruption in the higher fungus Ganoderma species. Process Biochem, 2017(56): 57-61.
[47] Chen B X, Wei T, Ye Z W. Efficient CRISPR-Cas9 gene disruption system in edible-medicinal mushroom Cordyceps militaris. Front Microbiol , 2018, doi. org/10. 3389/fmicb. 2018. 01157.
[48] Jiang W, Zhou H, Bi H. Demonstration of CRISPR-Cas9/sgRNA- mediated targeted gene modifcation in Arabidopsis, tobacco, sorghum and rice. Nucleic Acids Res, 2013, 41(20):e188.
[49] Jacobs T B, LaFayette P R, Schmitz R J. Targeted genome modifcations in soybean with CRISPR-Cas9. BMC Biotechnol, 2015, 15:16-25.
[50] Li Z, Liu Z B, Xing A. Cas9-guide RNA directed genome editing in soybean. Plant Physiol, 2015, 169(2):960-970.
[51] Brooks C, Nekrasov V, Lippman Z B. Efficient gene editing in tomato in the first generation using the clustered regularly interspaced short palindromic repeats/CRISPR associated 9 system. Plant Physiol. 2014, 166:1292-1297.
[52] Wang S, Zhang S, Wang W. Efficient targeted mutagenesis in potato by the CRISPR-Cas9 system. Plant Cell Rep, 2015, 34:1473-1476.
[53] Ren C, Liu X, Zhang Z. CRISPR-Cas9 mediated efficient targeted mutagenesis in chardonnay (Vitis vinifera L. ). Sci Rep, 2016, 6:32289.
[54] Kim H, Kim S T, Ryu J. CRISPR/Cpf1-mediated DNA-free plant genome editing. Nat Commun, 2017, 8: 14406-14412.
[55] Hu X, Wang C, Liu Q. Targeted mutagenesis in rice using CRISPR-Cpf1 system. J Genet Genomics, 2017, 44(1): 71-73.
[56] Shan Q, Wang Y, Li J. Targeted genome modifcation of crop plants using a CRISPR-Cas system. Nat Biotechnol, 2013, 31(8):686-688.
[57] Wang Y, Cheng X, Shan Q. Simultaneous editing of three homoeoalleles in hexaploid bread wheat confers heritable resistance to powdery mildew. Nat Biotechnol, 2014, 32(9):947-951.
[58] Mikami M, Toki S, Endo M. Parameters affecting frequency of CRISPR-Cas9 mediated targeted mutagenesis in rice. Plant Cell Rep, 2015, 34(10):1807-1815.
[59] Tang X, Levi G L. A CRISPR-Cpf1 system for efficient genome editing and transcriptional repression in plants. Nat Plants, 2017, 3, 17103.
[60] D’ Astolfo D S, Pagliero R J, Pras A. Efficient intracellular delivery of native proteins. Cell, 2015, 161(3): 674-690.
[61] Li D, Qiu Z, Shao Y. Heritable gene targeting in the mouse and rat using a CRISPR-Cas system. Nat Biotech, 2013, 31(8):681-683.
[62] Wang H, Yang H, Shivalila C. One-step generation of mice carrying mutations in multiple genes by CRISPR-Cas-mediated genome engineering. Cell, 2013, 153(4):910-918.
[63] Hwang W Y, Fu Y F, Reyon D. Efficient genome editing in zebrafish using a CRISPR-Cas system. Nat Biotech, 2013, 31(3):227-229.
[64] Friedland A E, Tzur Y B, Esvelt K M. Heritable genome editing in C. elegans via a CRISPR-Cas9 system. Nat Methods, 2013, 10(8):741-743.
[65] Niu Y, Shen B, Cui Y. Generation of gene-modified cynomolgus monkey via Cas9/RNA-mediated gene targeting in one-cell embryos. Cell, 2014, 156(4): 836-843.
[66] Xue W, Chen S D, Yin H. CRISPR-mediated direct mutation of cancer genes in the mouse liver. Nature, 2014, 514: 380-384.
[67] Ran F A, Hsu P D, Wright J. Genome engineering using the CRISPR-Cas9 system. Nat Protoc. 2013, 8(11):2281-2308.
[68] Tu M J, Lin L, Cheng Y L. A ‘new lease of life’: FnCpf1 possesses DNA cleavage activity for genome editing in human cells. Nucleic Acids Res, 2017, 45(19): 11295-11304.
[69] Zetsche, B. Heidenreich M, Mohanraju P. Multiplex gene editing by CRISPR-Cpf1 using a single crRNA array. Nat Biotech, 2017, 35: 31-34.
[70] Eszter T, Nora W, Petra B. Cpf1 nucleases demonstrate robust activity to induce DNA modification by exploiting homology directed repair pathways in mammalian cells. Biol Direct, 2016, 11, 46-69.
[71] Kleinstiver B P, Tsai S Q, Joung J K. Genome-wide specificities of CRISPR-Cas Cpf1 nucleases in human cells. Nat Biotech, 2016, 34: 869-874.
[72] Zhang Y, Long C Z, Li H. CRISPR-Cpf1 correction of muscular dystrophy mutations in human cardiomyocytes and mice. Sci Adv, 2017, 3: e1602814.
[73] Dong D, Ren K, Qiu X L, et al. The crystal structure of Cpf1 incomplex with CRISPR RNA. Nature, 2016, 532: 522-526.
[74] Zetsche B, Gootenberg J S, Abudayyeh O O. Cpf1 is a single RNA-guided endonuclease of a class 2 CRISPR-Cas system. Cell, 2015, 163(3): 759-771.
[75] Farzadfard F, Perli S D, Lu T K. Tunable and multifunctional eukaryotic transcription factors based on CRISPR-Cas. ACS Synth Biol, 2013, 2:604-613.
[76] Jusiak B, Cleto S, Perez-Pinera P. Engineering synthetic gene circuits in living cells with CRISPR technology. Trends Biotechnol, 2016, 34:535-547.
[77] Nissim L, Perli S D, Fridkin A. Multiplexed and programmable regulation of gene networks with an inte-grated RNA and CRISPR-Cas toolkit in Human Cells. Mol Cell, 2014, 54(4): 698- 710.
[78] Kearns N A, Pham H, Tabak B. Functional annotation of native enhancers with a Cas9-histone demethylase fusion. Nat Methods, 2015, 12: 401-403.
[79] Hong Y, Lu G Q, Duan J Z. Comparison and optimization of CRISPR/dCas9/gRNA genome- labeling systems for live cell imaging. Genome Biol, 2018, 19:39-49.
[80] Abudayyeh O O, Gootenberg J S, Essletzbichler P. RNA targeting with CRISPR-Cas13. Nature, 2017, 550(7675): 280-284.
[81] Konermann S, Lotfy P, Brideau N J. Transcriptome engineering with RNA-targeting type VI-D CRISPR effectors. Cell, 2018, 173(3): 665-676.
[82] East-Seletsky A, O’connell M R, Knight S C. Two distinct RNase activities of CRISPR-C2c2 enable guide RNA processing and RNA detection. Nature, 2016, 538(7624): 270-273。
[83] Grünewald J, Zhou R H, Garcia S P. Transcriptome-wide off-target RNA editing induced by CRISPR- guided DNA base editors. Nature, 2019, 10. 1038/s41586-019-1161-z.