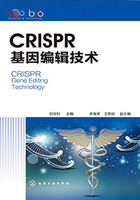
参考文献
[1] Capecchi M R. Gene targeting in mice: functional analysis of the mammalian genome for the twenty-first century. Nat Rev Genet, 2005, 6(6): 507-512.
[2] Smithies O, et al. Insertion of DNA sequences into the human chromosomal beta-globin locus by homologous recombination. Nature, 1985, 317(6034): 230-234.
[3] Evans M J, Kaufman M H. Establishment in culture of pluripotential cells from mouse embryos. Nature, 1981, 292(5819): 154-156.
[4] Bradley A, et al. Formation of germ-line chimaeras from embryo-derived teratocarcinoma cell lines. Nature, 1984, 309(5965): 255-256.
[5] Doetschman T, et al. Targetted correction of a mutant HPRT gene in mouse embryonic stem cells. Nature, 1987, 330(6148): 576-578.
[6] Thomas K R, Capecchi M R. Site-directed mutagenesis by gene targeting in mouse embryo-derived stem cells. Cell, 1987, 51(3): 503-512.
[7] Thompson S, et al. Germ line transmission and expression of a corrected HPRT gene produced by gene targeting in embryonic stem cells. Cell, 1989, 56(2): 313-321.
[8] Koller B H, et al. Germ-line transmission of a planned alteration made in a hypoxanthine phosphoribosyltransferase gene by homologous recombination in embryonic stem cells. Proc Natl Acad Sci U S A, 1989, 86(22): 8927-8931.
[9] Zijlstra M, et al. Germ-line transmission of a disrupted beta 2-microglobulin gene produced by homologous recombination in embryonic stem cells. Nature, 1989, 342(6248): 435-438.
[10] Thomas K R, Capecchi M R. Targeted disruption of the murine int-1 proto-oncogene resulting in severe abnormalities in midbrain and cerebellar development. Nature, 1990, 346(6287):847-850.
[11] MLA style: The Nobel Prize in Physiology or Medicine 2007, NobelPrize.org. Nobel Media AB 2019, Wed. 27 Nov 2019.; Available from: https://www.nobelprize.org/prizes/medicine/2007/7596-the-nobel-prize-in-physiology-or-medicine-2007/.
[12] Klug A. The discovery of zinc fingers and their development for practical applications in gene regulation and genome manipulation. Q Rev Biophys, 2010, 43(1): 1-21.
[13] Kim Y G, Cha J, Chandrasegaran S. Hybrid restriction enzymes: zinc finger fusions to Fok I cleavage domain. Proc Natl Acad Sci U S A, 1996, 93(3): 1156-1160.
[14] Boch J, Scholze H, Schornack S, et al. Breaking the code of DNA binding specificity of TAL-type Ⅲ effectors. Science, 2009, 326(5959): 1509-1512.
[15] Moscou M J, Bogdanove A J. A simple cipher governs DNA recognition by TAL effectors. Science, 2009, 326(5959): 1501.
[16] Ishino Y, et al. Nucleotide sequence of the iap gene, responsible for alkaline phosphatase isozyme conversion in Escherichia coli, and identification of the gene product. J Bacteriol, 1987, 169(12): 5429-5433.
[17] Groenen P M, et al. Nature of DNA polymorphism in the direct repeat cluster of Mycobacterium tuberculosis; application for strain differentiation by a novel typing method. Mol Microbiol, 1993, 10(5): 1057-1065.
[18] van Soolingen D, et al. Comparison of various repetitive DNA elements as genetic markers for strain differentiation and epidemiology of Mycobacterium tuberculosis. J Clin Microbiol, 1993, 31(8): 1987-1995.
[19] Mojica F J, Juez G, Rodriguez-Valera F. Transcription at different salinities of Haloferax mediterranei sequences adjacent to partially modified PstI sites. Mol Microbiol, 1993, 9(3): 613-621.
[20] Mojica F J, et al. Biological significance of a family of regularly spaced repeats in the genomes of Archaea, Bacteria and mitochondria. Mol Microbiol, 2000, 36(1): 244-246.
[21] Pourcel C, Salvignol G, Vergnaud G. CRISPR elements in Yersinia pestis acquire new repeats by preferential uptake of bacteriophage DNA, and provide additional tools for evolutionary studies. Microbiology, 2005, 151(Pt 3): 653-663.
[22] Mojica F J, et al. Intervening sequences of regularly spaced prokaryotic repeats derive from foreign genetic elements. J Mol Evol, 2005, 60(2): 174-182.
[23] Bolotin A, et al. Clustered regularly interspaced short palindrome repeats(CRISPRs)have spacers of extrachromosomal origin. Microbiology, 2005, 151(Pt 8): 2551-2561.
[24] Barrangou R, et al. CRISPR Provides Acquired Resistance Against Viruses in Prokaryotes. 2007, 315(5819): 1709-1712.
[25] Jinek M, et al. A programmable dual-RNA-guided DNA endonuclease in adaptive bacterial immunity. Science, 2012, 337(6096): 816-821.
[26] Jinek M, et al. RNA-programmed genome editing in human cells. Elife, 2013, 2: e00471.
[27] Mali P, et al. RNA-guided human genome engineering via Cas9. Science, 2013, 339(6121): 823-826.
[28] Cong L, et al. Multiplex genome engineering using CRISPR/Cas systems. Science, 2013, 339(6121): 819-823.
[29] Wang H, et al. One-step generation of mice carrying mutations in multiple genes by CRISPR/Cas-mediated genome engineering. Cell, 2013, 153(4): 910-918.
[30] Yang H, et al. One-step generation of mice carrying reporter and conditional alleles by CRISPR/Cas-mediated genome engineering. Cell, 2013, 154(6): 1370-1379.
[31] Komor A C, et al. Programmable editing of a target base in genomic DNA without double-stranded DNA cleavage. Nature, 2016, 533(7603): 420-424.
[32] Gaudelli N M, et al. Programmable base editing of A·T to G·C in genomic DNA without DNA cleavage. Nature, 2017, advance online publication.
[33] Anzalone A V, et al. Search-and-replace genome editing without double-strand breaks or donor DNA. Nature, 2019, 576: 149-157.
[34] Klompe S E, et al. Transposon-encoded CRISPR Cas systems direct RNA-guided DNA integration. Nature, 2019, 571(7764): 219-225.
[35] Strecker J, et al. RNA-guided DNA insertion with CRISPR-associated transposases. Science, 2019, 365(6448): 48-53.